In the verdant world of indoor gardening, where each leaf holds a story of resilience and growth, phosphorus plays a pivotal role. As an avid indoor gardener, I’ve delved deep into the mysteries of plant nutrition, and phosphorus deficiency is a common challenge that many enthusiasts encounter. In this comprehensive guide, I aim to shed light on the symptoms, causes, and remedies for phosphorus deficiency, drawing from both personal experience and scientific research.
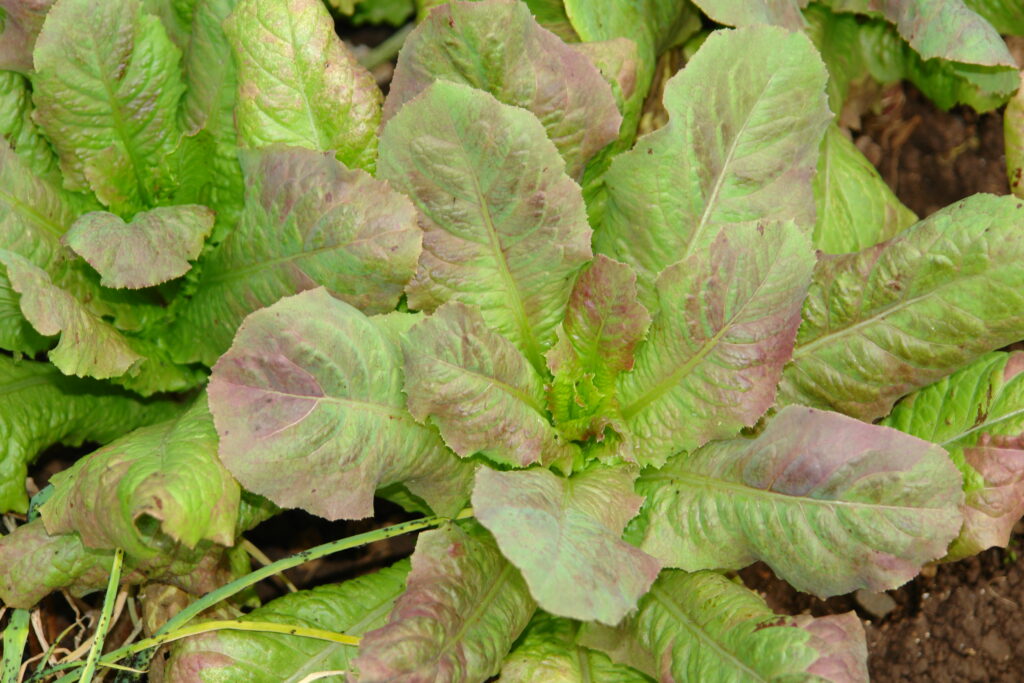
Stay Tuned For More Insightful Tips And Tricks On Indoor Plant Care In Future Blog Posts
Chapter 1: The Essence of Phosphorus :
Phosphorus is a crucial element in plant biology, playing multifaceted roles in various physiological processes. Understanding its significance unveils its pivotal contributions to energy transfer, biomolecule synthesis, and structural integrity.
Energy Transfer: Phosphorus is integral to ATP (adenosine triphosphate), the primary energy currency in cells. During photosynthesis, plants utilize light energy to convert carbon dioxide and water into glucose, a process that necessitates ATP. Phosphorus is a key component of the phosphate groups in ATP, facilitating the storage and transfer of energy within cells. Consequently, phosphorus deficiency can severely hamper ATP production, impairing vital cellular processes like growth and metabolism.
Synthesis of Biomolecules: Phosphorus is a fundamental constituent of various biomolecules essential for plant growth and development. In addition to ATP, phosphorus is a critical component of nucleic acids (DNA and RNA), phospholipids, and several coenzymes. In DNA and RNA, phosphate groups form the backbone, providing structural stability and facilitating the transmission of genetic information. Phospholipids, found in cell membranes, consist of phosphate groups that enable membrane formation and fluidity. Furthermore, phosphorus participates in the synthesis of coenzymes involved in diverse metabolic pathways, including those for carbohydrate and protein metabolism. Therefore, phosphorus deficiency can impair the synthesis of these biomolecules, adversely affecting plant growth and function.
Structural Component in Nucleic Acids and Membranes: Phosphorus contributes to the structural integrity of nucleic acids and membranes. In nucleic acids, phosphate groups link nucleotides together, forming the characteristic double helix structure of DNA and the single-stranded structure of RNA. This structural role is fundamental to the storage and expression of genetic information. Moreover, phospholipids, the primary constituents of cell membranes, contain hydrophilic phosphate heads and hydrophobic lipid tails. The arrangement of phospholipids forms the lipid bilayer, which serves as a selective barrier, regulating the passage of molecules in and out of cells. Thus, phosphorus deficiency can compromise the stability and function of nucleic acids and membranes, impacting various cellular processes and overall plant health
Chapter 2: Detecting the Deficiency :
Phosphorus deficiency in plants can lead to various symptoms, affecting their growth, development, and overall health. Here are some early signs and subtle cues indicating phosphorus deficiency, as well as visual symptoms manifesting in different plant parts:
Early Signs and Subtle Cues of Phosphorus Deficiency:
Stunted Growth: One of the earliest signs is reduced growth or stunted development. Plants lacking phosphorus may appear smaller than expected for their age.
Delayed Maturity: Phosphorus-deficient plants may take longer to mature compared to healthy plants of the same species.
Purple or Reddish Leaves: Some plants may exhibit a purple or reddish coloration in their leaves, particularly on the undersides. This is due to anthocyanin accumulation, which can be a response to phosphorus deficiency.
Dark Green Leaves: While it may seem contradictory, phosphorus deficiency can also cause dark green leaves, especially in some plants like corn. However, this green coloration may be accompanied by other symptoms.
Poor Flowering and Fruit Formation: Phosphorus deficiency can lead to reduced flowering and fruiting in flowering plants. Flowers may be smaller in size and number, and fruits may fail to develop properly.
Weak Root Development: Phosphorus is crucial for root growth and development. Deficient plants may exhibit poor root growth, with roots appearing small, thin, and underdeveloped.
Leaf Symptoms: Look for older leaves showing signs of yellowing, often starting at the tips and margins and progressing inward.
Visual Symptoms Manifesting in Different Plant Parts:
Leaves: Yellowing of older leaves, purple or reddish coloration on leaves, dark green leaves.
Stems and Petioles: Purplish or reddish discoloration, particularly in young stems and petioles.
Roots: Poor root development, reduced branching, and length, with roots appearing small and thin.
Flowers and Fruits: Reduced flowering and fruiting, smaller flowers, and poorly developed fruits.
Interpreting Soil Tests and Nutrient Analyses for Phosphorus Levels:
Soil Testing: Soil tests can provide valuable information about phosphorus levels in the soil. Typically, phosphorus levels are measured in parts per million (ppm) or milligrams per kilogram (mg/kg) of soil.
Interpretation: Phosphorus levels in soil are often interpreted based on established ranges for different crops. Optimal phosphorus levels can vary depending on the plant species and soil type. Soil test reports usually categorize phosphorus levels as low, medium, or high.
Factors Affecting Availability: It’s important to consider factors affecting phosphorus availability, such as soil pH, organic matter content, and soil temperature. Phosphorus availability tends to decrease in acidic soils and increase in alkaline soils.
Nutrient Analyses: In addition to soil tests, plant tissue analyses can also provide insights into phosphorus levels within the plant. Analyzing the phosphorus content in plant tissues can help diagnose deficiencies and guide fertilizer applications.
Fertilizer Recommendations: Based on soil test results and crop requirements, fertilizer recommendations can be tailored to address phosphorus deficiencies. Phosphorus-containing fertilizers, such as superphosphate or triple superphosphate, can be applied to replenish phosphorus levels in the soil and promote healthy plant growth.
Regular monitoring of plant health, coupled with soil testing and nutrient analyses, can help effectively manage phosphorus levels and ensure optimal plant nutrition.
Chapter 3: Above-Ground Manifestations :
The symptoms you provided could indicate several potential issues in plants, but they often point to a nutrient deficiency, particularly phosphorus. Here’s how each symptom aligns with this possibility:
Leaf Symptoms:
Stunted Growth: Phosphorus deficiency can hinder overall plant growth, leading to stunted development.
Dark Green or Purple Discoloration: This is a classic sign of phosphorus deficiency. The dark green coloration may occur initially, followed by purpling, especially on the underside of leaves.
Distorted Leaves: Phosphorus deficiency can cause leaves to become distorted, often appearing smaller than usual, with irregular shapes or margins.
Flower and Fruit Abnormalities:
Reduced Flowering: Phosphorus deficiency can lead to fewer flowers or a delay in flowering due to its role in energy transfer and flower development.
Poor Fruit Development: Since phosphorus is crucial for energy transfer and fruit formation, a deficiency can result in poorly developed or malformed fruits.
Premature Senescence: Phosphorus deficiency can trigger premature aging or senescence in plants, causing them to wilt and die earlier than expected.
Stem and Petiole Characteristics:
Purpling: Purpling of stems and petioles is another classic symptom of phosphorus deficiency. This purpling usually occurs due to the accumulation of anthocyanins, compounds responsible for the purple coloration, in response to low phosphorus levels.
Reduced Elongation: Phosphorus deficiency can inhibit cell elongation, leading to shorter stems and petioles.
To address these symptoms, you might consider:
- Testing the soil to confirm the deficiency and adjust fertilization accordingly.
- Applying phosphorus-rich fertilizers or amendments.
- Adjusting soil pH, as phosphorus availability can be influenced by soil pH levels.
- Ensuring proper drainage, as waterlogged soils can exacerbate phosphorus deficiency symptoms.
- Monitoring and adjusting irrigation practices to maintain optimal soil moisture levels.
- Providing adequate sunlight, as phosphorus uptake can be inhibited in low-light conditions.
If the issue persists despite corrective measures, it may be advisable to consult with a local agricultural extension service or a plant specialist for further diagnosis and treatment recommendations.
Chapter 4: Below-Ground Revelations :
Root Symptoms:
Root symptoms such as stunted growth, reduced branching, and increased susceptibility to pathogens are indicative of various issues within plants, often related to nutrient deficiencies or environmental stresses.
Stunted Growth: This occurs when plants fail to achieve their expected size for their age. It can result from a lack of essential nutrients, particularly nitrogen, phosphorus, or potassium, or from adverse environmental conditions like poor soil quality, inadequate water, or insufficient sunlight.
Reduced Branching: Reduced branching can also be a consequence of nutrient deficiencies, particularly nitrogen, which is crucial for vegetative growth and branching. It can also result from genetic factors or physical damage to the roots.
Increased Susceptibility to Pathogens: When plants are stressed due to nutrient deficiencies or environmental factors, their immune systems may weaken, making them more susceptible to attacks by pathogens such as fungi, bacteria, or viruses. This vulnerability can lead to various diseases that further impair plant growth and productivity.
Mycorrhizal Associations and Phosphorus Uptake:
Mycorrhizal associations are symbiotic relationships between plant roots and fungi. These associations are widespread in nature and can significantly impact plant nutrient uptake, particularly phosphorus.
Arbuscular Mycorrhizal (AM) Fungi: These fungi form symbiotic relationships with the roots of most plant species. The fungal hyphae extend into the soil, greatly increasing the surface area for nutrient absorption, including phosphorus. In return, the fungi receive sugars and other organic compounds from the plant.
Phosphorus Uptake: Phosphorus is often present in soils in forms that are not readily available to plants. Mycorrhizal fungi can release enzymes that break down organic phosphorus compounds, making phosphorus more accessible to the plant. Additionally, the fungal hyphae can explore a larger volume of soil than plant roots alone, increasing the likelihood of encountering phosphorus-rich patches.
Rhizosphere Dynamics and Phosphorus Availability:
The rhizosphere is the region of soil influenced by root activity, including the exudation of compounds by roots and the interactions between roots, soil, microorganisms, and nutrients like phosphorus.
Root Exudates: Plants release a variety of organic compounds into the soil through their roots. These exudates can include sugars, amino acids, organic acids, and enzymes. Some of these compounds can stimulate the growth of beneficial microorganisms that enhance phosphorus availability.
Microbial Activity: Microorganisms in the rhizosphere play a crucial role in nutrient cycling, including phosphorus. Certain bacteria and fungi can solubilize phosphorus from organic and mineral forms, making it more accessible to plants. Additionally, some microbes form symbiotic relationships with plants, such as mycorrhizal fungi, which enhance phosphorus uptake as discussed earlier.
Soil pH and Phosphorus Availability: Soil pH influences the availability of phosphorus to plants. In acidic soils, phosphorus tends to be more tightly bound to soil particles and less available to plants, whereas in alkaline soils, phosphorus may precipitate and become unavailable. Rhizosphere dynamics can affect soil pH through the release of organic acids by plant roots, which can help solubilize phosphorus and increase its availability.
Understanding these interactions between roots, soil, microorganisms, and nutrients like phosphorus is essential for optimizing plant growth and nutrient uptake in agricultural and ecological systems.
Chapter 5: Physiological Consequences :
Impaired photosynthesis and reduced carbon assimilation: Photosynthesis is the process by which plants convert light energy into chemical energy, synthesizing glucose from carbon dioxide and water. Impairment in this process can occur due to factors like inadequate light, damage to chloroplasts (the site of photosynthesis), or a deficiency in essential nutrients. As a result, the plant’s ability to produce carbohydrates, vital for growth and energy, is hindered, leading to stunted growth and reduced vigor.
Altered nutrient uptake and transport mechanisms: Nutrient uptake and transport are crucial processes for a plant’s growth and development. Various factors, such as soil conditions, pH levels, and the presence of toxins or pollutants, can disrupt these mechanisms. When nutrient uptake and transport are compromised, the plant may suffer from deficiencies in essential elements like nitrogen, phosphorus, potassium, and micronutrients. This can manifest as yellowing of leaves (chlorosis), poor flowering or fruiting, and overall diminished health.
Susceptibility to biotic and abiotic stresses due to compromised defense mechanisms: Plants have evolved intricate defense mechanisms to protect themselves against both biotic (e.g., pests, pathogens) and abiotic (e.g., drought, salinity, extreme temperatures) stresses. However, when their normal physiological functions are impaired, these defense mechanisms may become compromised. For instance, a weakened plant may produce fewer defensive compounds or have a slower response to stress signals, making it more susceptible to damage from pests, diseases, or adverse environmental conditions.
Chapter 6: Environmental and Soil Factors :
pH Levels and Phosphorus Availability:
- pH refers to the measure of acidity or alkalinity of a substance, such as soil. It ranges from 0 to 14, with 7 being neutral. Values below 7 are acidic, and above 7 are alkaline.
- Phosphorus availability is greatly influenced by soil pH.
- In acidic soils (pH below 7), phosphorus tends to be less available because it reacts with aluminum and iron, forming insoluble compounds that plants cannot absorb effectively.
- Conversely, in alkaline soils (pH above 7), phosphorus availability can also be reduced due to the formation of insoluble calcium phosphates.
- The optimal pH range for phosphorus availability is generally considered to be between 6.0 and 7.5, although this can vary depending on the specific plant species.
Soil Texture and Structure Influencing Phosphorus Retention and Release:
- Soil texture refers to the composition of soil particles, including sand, silt, and clay.
- Soil structure refers to the arrangement of soil particles and the spaces between them.
- Sandy soils have larger particles and larger pore spaces, which allow for faster water drainage but can lead to faster leaching of phosphorus.
- Clayey soils have smaller particles and smaller pore spaces, which can lead to greater retention of phosphorus but may also result in slower nutrient release to plants.
- Organic matter in soil can also influence phosphorus availability. Organic matter acts as a reservoir for phosphorus, releasing it slowly over time as it decomposes.
Waterlogged Conditions and Their Effect on Phosphorus Uptake:
- Waterlogged or water-saturated conditions can have both positive and negative effects on phosphorus uptake by plants.
- In waterlogged conditions, soil becomes anaerobic (lacking oxygen), which can inhibit the activity of soil microbes responsible for releasing phosphorus from organic matter.
- On the other hand, waterlogged conditions can also reduce phosphorus fixation by iron and aluminum oxides in acidic soils, making phosphorus more available to plants.
- However, prolonged waterlogging can lead to nutrient imbalances and root damage in plants, ultimately affecting their ability to take up phosphorus efficiently.
Chapter 7: Management Strategies :
Soil amendment techniques such as phosphorus fertilization and organic matter incorporation play vital roles in enhancing soil fertility and crop productivity. Here’s a breakdown of each:
- Phosphorus Fertilization:
- Phosphorus (P) is a crucial nutrient for plant growth, playing a key role in energy transfer, photosynthesis, and root development.
- Phosphorus fertilizers are commonly applied to soils deficient in phosphorus to boost plant growth and yield.
- Different forms of phosphorus fertilizers include rock phosphate, triple superphosphate, and monoammonium phosphate, among others.
- Proper application rates and timing are essential to prevent over-application and minimize environmental pollution, such as phosphorus runoff into water bodies, which can lead to eutrophication.
- Techniques like precision agriculture help optimize phosphorus fertilizer application by using data-driven approaches to apply the right amount of fertilizer at the right time and place, reducing waste and environmental impact.
- Organic Matter Incorporation:
- Organic matter serves as a reservoir of nutrients, including phosphorus, and improves soil structure, water retention, and microbial activity.
- Incorporating organic materials such as compost, manure, crop residues, and cover crops into the soil enhances soil fertility and promotes sustainable agriculture.
- Organic matter incorporation increases phosphorus availability to plants by releasing bound phosphorus through decomposition and mineralization processes.
- No-till and reduced tillage practices help preserve soil organic matter by minimizing soil disturbance and erosion, thus promoting long-term soil health and fertility.
Biofortification and genetic engineering approaches aim to improve phosphorus use efficiency in crops:
- Biofortification:
- Biofortification involves breeding or selecting crops with enhanced nutrient content, including phosphorus.Through conventional breeding techniques or genetic modification, crops can be developed to accumulate more phosphorus in edible plant parts.
- Biofortified crops contribute to addressing malnutrition and nutrient deficiencies in populations reliant on staple crops for their diet.
- Genetic Engineering:
- Genetic engineering techniques enable the manipulation of plant genes to enhance traits such as phosphorus uptake, utilization, and tolerance.
- Researchers can identify and introduce genes responsible for phosphorus transporters, enzymes involved in phosphorus metabolism, or traits conferring phosphorus efficiency from wild or genetically diverse plant species into cultivated crops.
- Genetically engineered crops with improved phosphorus use efficiency can thrive in low-phosphorus soils, reducing the need for phosphorus fertilizers and promoting sustainable agriculture.
Sustainable agricultural practices promoting phosphorus recycling and conservation include:
- Phosphorus Recycling:
- Recycling organic waste materials like compost, manure, and crop residues reintroduces phosphorus and other nutrients back into agricultural soils.
- Technologies such as anaerobic digestion and composting convert organic waste into nutrient-rich soil amendments, reducing the reliance on synthetic fertilizers and minimizing waste disposal issues.
- Conservation Tillage:
- Conservation tillage practices, including no-till and reduced tillage, minimize soil disturbance and erosion, preserving soil organic matter and nutrient content, including phosphorus.
- These practices help maintain soil structure and fertility, reduce nutrient runoff, and mitigate soil erosion, promoting long-term sustainability in agricultural systems.
- Cover Cropping:
- Cover crops, such as legumes and grasses, are planted during fallow periods to cover and protect the soil surface, reduce erosion, and improve soil health.
- Leguminous cover crops can fix atmospheric nitrogen, reducing the need for nitrogen fertilizers, and contribute to phosphorus cycling and availability in the soil through root exudation and decomposition.
Chapter 8: Case Studies and Success Stories :
Real-world examples illustrating the diagnosis and management of phosphorus deficiency:
- Agricultural Farming in the Midwest, USA:
- Diagnosis: Farmers often use soil testing to detect phosphorus deficiency. For example, if soil tests reveal low phosphorus levels, coupled with symptoms like stunted growth and purpling of leaves in crops like corn and soybeans, phosphorus deficiency is suspected.
- Management: Farmers apply phosphorus fertilizers based on soil test recommendations. This could involve using fertilizers like diammonium phosphate (DAP) or triple superphosphate (TSP) to replenish phosphorus levels in the soil and ensure optimal crop growth.
- Rice Farming in Southeast Asia:
- Diagnosis: Farmers observe symptoms like delayed flowering, reduced tillering , and poor root development in rice paddies, indicating phosphorus deficiency.
- Management: Farmers adopt practices like incorporating organic matter into the soil, utilizing phosphorus-containing organic fertilizers such as compost or manure, and implementing crop rotation with leguminous plants to fix atmospheric nitrogen and enhance phosphorus availability.
Crop-specific strategies tailored to address phosphorus deficiency in diverse agroecosystems:
- Maize (Corn) Farming in Sub-Saharan Africa:
- Strategy: Farmers employ agronomic practices like intercropping maize with legumes such as cowpea or soybean. Legumes have symbiotic relationships with nitrogen-fixing bacteria that enhance soil fertility, including phosphorus availability. Additionally, using phosphorus-efficient maize varieties developed through breeding programs can optimize phosphorus uptake and utilization.
- Wheat Cultivation in Australia:
- Strategy: Australian wheat farmers utilize precision agriculture techniques to manage phosphorus deficiency. This involves soil mapping to identify areas with low phosphorus levels, followed by targeted application of phosphorus fertilizers using variable rate technology (VRT). By adjusting fertilizer rates based on spatial variability in soil phosphorus, farmers can optimize phosphorus use efficiency and minimize environmental impacts.
- Coffee Plantations in Latin America:
- Strategy: Coffee growers in regions like Colombia and Brazil face phosphorus deficiency due to acidic soils and intensive cultivation. To address this, farmers incorporate lime to raise soil pH and reduce phosphorus fixation. They also adopt practices like agroforestry, planting shade trees alongside coffee plants, which not only improve soil structure but also enhance phosphorus availability through organic matter decomposition and root exudation.
Chapter 9: Future Perspectives and Innovations :
Advances in phosphorus research have become increasingly critical due to the essential role phosphorus plays in agricultural productivity, environmental sustainability, and global food security. Here’s a breakdown of the key areas of advancement:
- Nanotechnology in Phosphorus Management: Nanotechnology offers promising solutions for efficient phosphorus management in agriculture. Nanostructured materials, such as nano-sized phosphorus fertilizers and nanosensors, can enhance phosphorus uptake by plants, reduce nutrient losses through leaching and runoff, and improve fertilizer use efficiency. Additionally, nanotechnology enables controlled release of phosphorus, ensuring its availability to crops over an extended period.
- Precision Agriculture and Digital Farming: Precision agriculture, aided by digital farming technologies, allows farmers to optimize phosphorus application by precisely matching nutrient inputs to crop requirements. Satellite imagery, drones, soil sensors, and data analytics help monitor soil phosphorus levels, crop growth, and environmental conditions in real-time, enabling targeted fertilization strategies. Digital platforms and mobile apps provide farmers with personalized recommendations for phosphorus management, considering factors such as soil type, crop variety, and weather patterns.
- Harnessing Microbial Communities: Microorganisms play a crucial role in phosphorus cycling and plant nutrition. Research focuses on harnessing microbial communities, particularly phosphate-solubilizing bacteria and mycorrhizal fungi, to enhance phosphorus mobilization and plant growth promotion. Biofertilizers containing beneficial microbes can improve phosphorus availability in soils, reduce the reliance on chemical fertilizers, and promote sustainable agricultural practices. Moreover, microbial inoculants tailored to specific soil conditions and crop requirements offer customized solutions for phosphorus management.
- Policy Implications and Global Initiatives: Sustainable phosphorus management requires coordinated efforts at the policy and international levels. Policymakers are increasingly recognizing the importance of phosphorus stewardship in achieving food security, mitigating environmental pollution, and addressing global challenges such as climate change. Initiatives like the Global Phosphorus Research Initiative (GPRI) and the Sustainable Phosphorus Alliance advocate for sustainable phosphorus use, recycling, and conservation. Policy measures may include promoting nutrient management planning, regulating phosphorus runoff from agricultural and urban sources, incentivizing phosphorus recycling from organic wastes, and fostering international collaborations for knowledge sharing and capacity building.
By integrating nanotechnology, precision agriculture, microbial symbiosis, and policy frameworks, researchers and stakeholders can advance sustainable phosphorus management practices to ensure food security, environmental protection, and agricultural resilience in the face of evolving challenges.
In the intricate tapestry of plant health, phosphorus deficiency emerges as a critical thread, demanding attention and action. By deciphering the subtle signs and symptoms, we empower ourselves to nurture thriving plant communities and sustain agricultural productivity. As we navigate the complexities of phosphorus dynamics, let us embrace innovation and collaboration to cultivate a greener, more resilient future for generations to come.